ADVERTISEMENT
Ovine Forestomach Matrix in the Surgical Management of Complex Volumetric Soft Tissue Defects: A Retrospective Pilot Case Series
© 2023 HMP Global. All Rights Reserved.
Any views and opinions expressed are those of the author(s) and/or participants and do not necessarily reflect the views, policy, or position of ePlasty or HMP Global, their employees, and affiliates.
Abstract
Background. Volumetric soft tissue loss is an urgent surgical issue and can frequently lead to suboptimal outcomes for patients due to significant soft tissue loss, compromised vital structures, and contamination. Ovine forestomach matrix (OFM) has demonstrated clinical success in the surgical management of soft tissue defects, especially in contaminated fields, and provides an effective option for immediate coverage of exposed vital structures before definitive closure.
Methods. This retrospective pilot case series (n = 13 defects) evaluated the clinical effectiveness of OFM (graft and/or particulate formats) in the surgical management of contaminated volumetric soft tissue defects. Patients presented with significant soft tissue loss, often with exposed viscera, tendon, bone, or muscle, and were treated with OFM as part of their inpatient surgical management. All patients had at least 1 significant comorbidity with the potential to complicate their healing trajectory. The primary study endpoint was time to 100% granulation tissue coverage (days), and the secondary endpoint was any device-related postoperative complications.
Results. A total of 13 volumetric soft tissue defects were evaluated in 10 patients who underwent surgical reconstruction. Mean defect age was 3.5 ± 5.6 weeks, and mean area was 217.3 ± 77.9 cm2. Most defects had exposed structures (85%), and all defects were Centers for Disease Control and Prevention grade 2 or higher. Mean time to 100% granulation tissue formation was 23.4 ± 9.2 days, with a median product application of 1.0. Staged reconstruction was used in 7 of 13 defects, with the remainder (6 of 13) left to heal via secondary intention using standard wound care protocols. There were no major postoperative infections or adverse events (mean follow-up, 7.4 ± 2.4 weeks.)
Conclusions. This retrospective pilot case series builds on a growing body of evidence that OFM can be utilized to facilitate the formation of functional, well-vascularized soft tissue in large contaminated volumetric soft tissue defects.
Introduction
Complex soft tissue defects requiring reconstruction can be the result of a wide variety of etiologies, including necrotizing soft tissue infections (NSTIs), surgical dehiscence, burns, trauma, pressure injuries, and other etiologies. The resulting defect may heal by secondary intention but with relatively lengthy healing times involving frequent dressing changes, all while being prone to infection.1 Surgical management of these full-thickness defects can vary widely and may include immediate coverage with a split-thickness skin graft (STSG), use of local or autologous free flaps, use of dermal matrices, and/or negative pressure wound therapy (NPWT). The current definition of a full-thickness wound is a defect that extends through the dermis, often with exposed structures (eg, bone, tendon, fascia, or muscle).2 Often in trauma and acute care, full-thickness defects are relatively deep with loss of the dermal layer as well as the deeper subcutaneous tissue layers (eg, adipose, fascia, or muscle). To better classify the severity and depth of these defects, we propose an additional definition for these large 3-dimensional complex wounds, referring to these wounds collectively as “volumetric soft tissue defects.” This proposed terminology draws from the field of civilian and combat-related trauma where the term “volumetric muscle loss” (VML) is used to define significant loss of the musculature.3 We propose that this terminology allows for a better visualization of the challenge in successfully reconstructing these large-volume, full-thickness dermal defects.
Initially, the primary goal of the reconstruction of a full-thickness wound is immediate coverage of exposed vital structures, such as viscera, bone, tendon, or fascia. This may be achieved via placement of a STSG, which decreases the potential for infection,4 facilitates closure when healing by primary or secondary intention is not feasible,5 and provides immediate coverage of any exposed structures.6 However, in doing so, patients can be left with irregular scars that have significant differences in contour between the uninjured skin and the healed defect. In cosmetic surgery, this contour defect is often referred to as a “step-off” deformity.7 This is exacerbated in volumetric soft tissue defects due to their depth, such that immediate application of a STSG in this scenario may lead to a step-off deformity. An alternate reconstructive approach to full-thickness and volumetric defects is a free flap procedure. However, a free flap procedure may result in overly prominent tissue requiring subsequent debulking procedures.8 Additionally, volumetric soft tissue defects are not always candidates for local or free tissue flaps as they tend to have undermining sinus tracts and/or an irregular wound surface that can compromise the arterial blood flow necessary to facilitate flap success.9
Modern dermal matrices are now very much part of the reconstructive ladder and may provide an alternative to, or augment, more complex tissue transfer procedures. In some instances, dermal matrices may be deployed in the first instance so that more complex procedures can be held as a back-up to these local less invasive matrix-based approaches.10 Dermal matrices include a variety of technologies, both synthetically manufactured or biologic, being derived from mammalian tissue extracellular matrix (ECM). ECM-based products are a good option in contaminated fields since typically synthetic dermal matrices are contraindicated for this environment as they may serve as a nidus for bacterial growth.11 In contrast, ECM-based dermal matrices are generally tolerant of a contaminated surgical site.12 For example, Ousey et al13 have proposed that dermal matrices may be deployed in Centers for Disease Control and Prevention (CDC) grade 2 and grade 3 defects following adequate sharp debridement. As it relates to volumetric soft tissue defects, dermal matrices provide a robust option to traditional tissue transfer procedures (ie, STSG and free or rotational flaps) as they enable a staged approach to reconstruction, providing immediate coverage of exposed structures and tissue infill to enable contour restoration of these deep and typically irregular defects.
Ovine forestomach matrix (OFM) is a decellularized ECM biomaterial that has been fabricated into a variety of devices and utilized for soft tissue regeneration in a range of contaminated defects, including hidradenitis suppurativa,14 pilonidal sinus,15 chronic diabetic foot ulcers,16 chest wall reconstruction,17 and abdominal wall repair.18-24 The success of OFM in these reconstructions that involve contaminated fields can be attributed to its ability to form well-vascularized tissue25 while modulating wound proteases that are known to prolong inflammation.26 This, in theory, should allow the patient’s immune system to primarily fend off persistent microbial contamination and progress the wound to an accelerated closure.27
In this retrospective pilot case series, we report our initial experience using OFM-based devices in conjunction with NPWT to provide coverage and tissue infill in volumetric soft tissue defects.
Materials and Methods
General
The retrospective study included patients who had undergone surgical reconstruction during the period January 2021 to February 2023 at a single facility. All patients provided written informed consent for their images and data to be used for research and publication purposes. The retrospective study was reviewed, and ethical oversight waived by WCG Clinical institutional review board (Puyallup, WA). The study was conducted in accordance with World Medical Association Declaration of Helsinki ethical guidelines. Patient demographics (eg, age, gender, significant baseline comorbidities), defect etiology and characteristics (eg, size, CDC grade), and outcomes (eg, 100% granulation tissue formation, recurrence, complications) were captured in Excel (Microsoft Corporation). The primary study outcome was defined as time (days) for complete graft integration and volumetric fill of the soft tissue defect with granulation tissue. Secondary endpoints included postoperative complications (eg, infection, pain, and recurrence). Descriptive statistics (eg, median, mean, SD) were computed using Excel.
Surgical Reconstruction
OFMs in either graft (Myriad Matrix Soft Tissue Bioscaffold; Aroa Biosurgery Limited) or morselized (particulate or powder) format (Myriad Morcells; Aroa Biosurgery Limited) were used according to the instructions for use. Patients were given general anesthesia, the surgical site was prepared with povidone-iodine (Betadine; Cumulus Pharmaceutical LLC), and the patient was surgically draped. The defects were thoroughly debrided to remove all necrotic tissue and lavaged with sterile saline. Defect dimensions and depth were recorded with a surgical ruler post debridement. Utilization of either the OFM graft (3- or 5-layer), morselized OFM, or a combination of the 2 products was based on clinical judgment of the attending surgeon. The OFM devices were rehydrated (<5 min, sterile saline), trimmed to size as required, and fixed to the defect edges with either suture or staples. The grafts were dressed with a nonadherent layer (Xeroform; McKesson Medical-Surgical), then NPWT interface black foam and the NPWT system (V.A.C. Therapy; 3M/KCI). NPWT systems were set to 125 mm Hg, and dressings were changed every 5 to 7 days. At dressing change, the defects were assessed for integration of the OFM graft and any complications. Complete graft integration and percentage granulation tissue formation was judged by the surgical team at the time of dressing change. At the discretion of the surgical team, definitive closure of the defects was achieved via STSG or secondary intention, according to institutional protocols.
Results
Ten consecutive participants were included in the study who had presented with a total of 13 soft tissue defects at a single facility and had undergone surgical reconstruction with an OFM graft (Table 1). Mean participant age was 52.6 ± 14.2 years; 5 were female, 4 were male, and 1 was transgender (male-to-female). All participants except one presented with significant complicating comorbidities (Table 1). Surgical wound dehiscence (SWD) (23%) and trauma (38%) were the primary causes of the volumetric defects; 2 others were pressure injuries, and 1 arose from a surgical intervention (compartment syndrome). Overall mean defect age was 3.5 ± 5.6 weeks. Exposed structures (eg, bone, viscera) were present in 86% of the defects, and all defects were either CDC grades 2 (62%) or 3 (38%). The mean defect area was 217.3 ± 77.9 cm2 with a mean depth of 3.2±5.2 cm.
Three defects were managed with the OFM matrix graft only, 4 received the OFM as a morselized format, and 6 defects received both, whereby the morselized graft was first applied to the wound bed, then the matrix graft was applied (Table 2). Both formats of the OFM graft were easy to deploy as part of the surgical procedure and could be trimmed to size and secured with sutures or staples as needed. The median product application was 1.0 across all defects. All defects received NPWT in conjunction with the OFM graft, with a mean duration of NPWT usage of 3.8 ± 1.3 weeks (Table 2). In all cases, the OFM grafts integrated into the regenerating tissue, with a mean time to complete integration of the graft and 100% granulation tissue of 23.4 ± 9.2 days.
Seven defects (54%) had a staged reconstruction in which STSG was used for definitive closure. Of those participants who received a STSG, the median STSG take at 1 week was 100%. Five of the 10 participants were lost to follow-up before complete healing, and the mean time to closure of defects in the remaining patients was 6.4 ± 2.8 weeks. There was one minor wound dehiscence reported (participant #1), but otherwise there were no infections, seromas, or other complications during the postoperative follow-up period (mean, 7.4 ± 2.4 weeks)(Table 2).
The following sections highlight 3 sample cases from the study data and do not reflect the chronological order of cases.
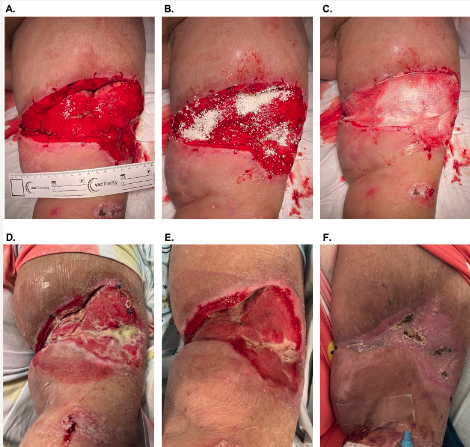
Case 1
A 61-year-old female patient (participant 2 ; Table 1) with morbid obesity and hypertension presented for follow-up evaluation of multiple soft tissue traumas sustained in a high-velocity motor vehicle accident, including Morel-Lavallee lesions of the left arm (Figure 1) and left leg (not shown). The patient had undergone multiple debridement procedures to both defects, and closure of the leg defect was attempted via placement of STSG that ultimately failed.
The patient was scheduled to undergo simultaneous reconstruction of the arm and leg defects with OFM. Before application of the OFM, the defects were irrigated with chlorhexidine solution, 50/50 povidone-iodine, and saline. After debridement, the arm defect measured 19 cm × 10 cm × 4 cm (Figure 1A). Morselized OFM was applied dry and rehydrated in situ with sterile saline and blood (Figure 1B). An OFM graft (10 cm × 20 cm, 5-layer) was rehydrated with saline, trimmed to size, then placed on top of the morselized OFM and secured to the defect (Figure 1C). The defects were dressed with an occlusive petrolatum dressing (Xeroform; Curad Medical), and a standard NPWT was placed at 125 mm Hg. NPWT was used for 3 weeks, with weekly dressing changes. Complete integration of the OFM graft and 100% granulation tissue over the exposed tendon was achieved in 21 days with the regenerated tissue being flush to the level of adjacent skin (Figure 1E). Definitive closure was achieved via application of a STSG at day 20, with 100% take of STSG after 1 week. At postoperative day 42, there was functional soft tissue with no complications (Figure 1F).
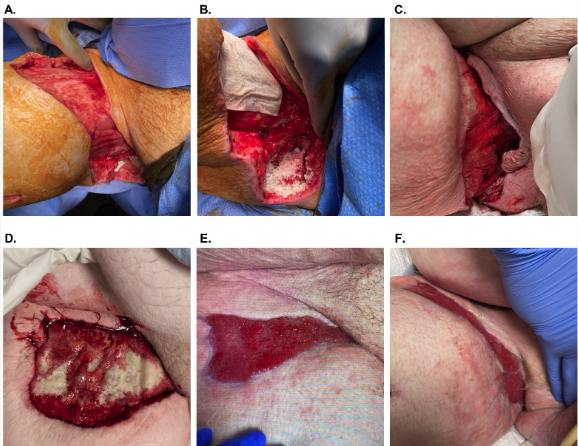
Case 2
A 37-year-old male patient (participant 5) with obesity and spina bifida presented with an NSTI (Fornier’s gangrene) of the right groin with exposed tendon and muscle (Figure 2). The patient had been admitted to the hospital the previous week and undergone multiple debridements and had subsequently been discharged to long-term acute care facility with standard NPWT (125 mm Hg). As the condition of the soft tissue defect continued to deteriorate, the patient was readmitted to the hospital for surgical intervention.
Upon readmission, the patient underwent surgical debridement and irrigation (chlorhexidine solution, 50/50 povidone-iodine, and saline) of the defect. After debridement, the defect measured 30 cm × 10 cm × 3 cm (Figure 2A). Morselized OFM was applied dry to the deepest and most irregular parts of the defect and rehydrated in situ with saline (Figure 2B). Two OFM grafts (10 cm × 20 cm, 5-layer) were rehydrated (saline), quilted together with absorbable polyglycolic acid suture to create a single larger graft, and then trimmed to the size of the defect. The OFM graft was applied over the morselized OFM and secured in the defect with staples (Figure 2C). The defect was dressed with a nonadherent layer and NPWT (125 mm Hg) as previously described. NPWT was continued for 4 weeks with weekly NPWT changes. At postoperative day 43 (Figure 2E), there was complete tissue coverage over the exposed bone and tendon and contour restoration. Standard wound care was initiated to close via secondary intention, and the participant was lost to follow-up after week 8.
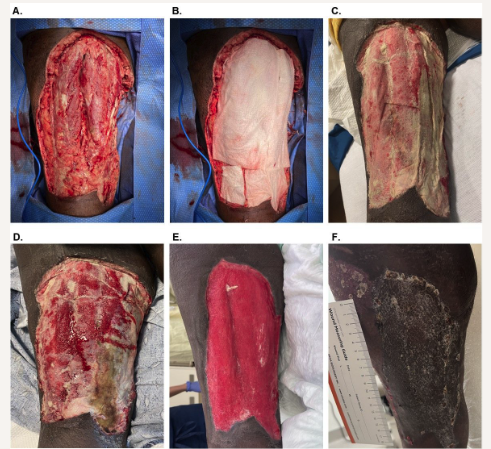
Case 3
A 56-year-old transgender (male-to-female) patient (participant 6) with medical history significant for HIV infection and hypertension was experiencing homelessness and developed an NSTI of the posterior left thigh. The participant presented to the emergency department with worsening pain and swelling and was immediately admitted for surgical debridement of necrotic and infectious tissue. The patient was managed acutely by another health care professional, undergoing multiple surgical debridements over the course of several days before leaving against medical advice.
Subsequently, the patient presented to the authors’ facility approximately 2 weeks after the initial admission. The patient was taken to surgery and underwent a sharp debridement, resulting in a defect measuring 21 cm × 10 cm × 2 cm (Figure 3A). Two OFM grafts (10 cm × 20 cm, 5-layer) were rehydrated (saline), trimmed, placed into the defect, and secured with absorbable sutures (Figure 3B). The graft was dressed with an occlusive petrolatum dressing (Xeroform; Curad Medical) and standard NPWT (125 mm Hg). The patient underwent dressing changes every 5 to 7 days whereby the NPWT interface foam was replaced, but the occlusive dressing was left in place so as to not disturb the underlying graft. At postoperative day 16, the graft was well adhered and had approximately 70% graft integration (Figure 3D). There was no sign of infection or complication, and NPWT was continued for an additional 12 days. By postoperative day 28, the defect’s depth had significantly reduced such that the regenerating tissue was now approximately planar to the surrounding intact skin (Figure 3E), and a STSG was applied. At 1 week after STSG application, there was 100% graft take with no complications noted. Three weeks later, the defect was fully epithelialized with good tissue pliability (Figure 3F).
Discussion
Full-thickness wounds are commonly categorized and studied based upon wound etiology (eg, full-thickness burn, stage IV pressure injury, NSTI, and others). To the authors’ knowledge, there is no existing term to group soft tissue defects based on wound bed complexity and depth regardless of the cause of initial defect. This void in nomenclature has prompted the authors to define the term “volumetric soft tissue defect” as a means to unify wounds of varying etiologies that share common features of irregular wound surface, depth that often includes the subcutaneous layers, exposed vital structures, and the high propensity for bacterial contamination (CDC grade 2 or higher).
The challenges with reconstruction of large volumetric soft tissue defects include the relative scale of these defects (depth and surface area); irregularity of the defect surface that may include undermining; presence of exposed vital structures that are prone to desiccation or necrosis; and frequent microbial contamination. Achieving rapid tissue infill of these defects and coverage of any exposed vital structures is the primary goal of reconstruction and has traditionally been approached with autologous tissue flaps. However, due to comorbidities, not all patients are suitable candidates for large autologous flap procedures,28 and often the surgical complexity of autologous tissue transfers brings a new set of challenges. Dermal matrices, like OFMs, are now an accepted part of the reconstructive ladder and facilitate the patient’s own body to form a functional layer of well-vascularized tissue to provide coverage and fill the soft tissue defect. The use of dermal matrices was first proposed by Yannas and Burke,29,30 who demonstrated clinically the use of a synthetic bilayer dermal matrix where the “artificial dermis resembles normal dermis and serves as a template for the synthesis of new connective tissue and the formation of a ‘neodermis,’ while it is slowly biodegraded.” The development of dermal matrices enabled the advent of staged reconstructions to expedite definitive closure of complex tissue defects. Since these early studies the range of dermal matrices has ever expanded with new synthetic and naturally derived matrices being commercialized and adopted into clinical use. However, not all dermal matrices are the same with respect to clinical performance. For example, some synthetic dermal matrices are prone to infection or are relatively slow to integrate into the regenerating neodermis.31 A subset of the available dermal matrices are naturally derived from human or animal tissue ECM. A key differential between ECM-based dermal matrices and their synthetic counterparts is a composition that includes naturally occurring proteins that exist in abundance in all soft tissues and are known to play key roles in soft tissue regeneration. ECM-based dermal matrices are known to rapidly vascularize and integrate into the regenerating neodermis and over time undergo a process of remodeling, mimicking the natural remodeling of ECM that occurs in all tissues.32
In this retrospective case series, there were a variety of volumetric soft tissue defects that included NSTIs, acute traumatic soft tissue injuries, and surgical wound dehiscence. There was a total of 13 defects included in this retrospective review where OFM matrix and/or morselized OFM were used to develop a healthy neodermis. In all cases, the defects were contaminated (CDC grade 2, or higher), and in 11 of the 13 cases, there was an exposed structure, including bone, surgical mesh, tendon, or viscera. The median time to complete integration of the graft and 100% granulation tissue formation was 21 days (mean, 23.4 ± 9.2 days). In 11 of the 13 defects, only a single application of OFM was required to regenerate a healthy neodermis. This is in contrast to the other available ECM-based surgical grafts where repeat applications are often necessary to achieve tissue infill of deep defects.33,34
One key goal of utilizing OFM in these volumetric defects is to restore the tissue depth and contour as close to anatomic normalcy as possible, as seen in Figures 2E and 3E. This contour restoration can aid in maximizing function and cosmetic outcomes. Concurrent NPWT usage ranged from 3 to 8 weeks with a median of 4 weeks. Importantly, the authors found that the frequency of dressing changes (5-7 days) was reduced relative to the traditional frequency of 3 times per week.35 This observation may have significant long-term impacts on the health economics of managing these complex defects by reducing the burden and costs associated with postoperative care of these patients. While reported costs vary, one publication estimated the mean theoretical cost of standard NPWT dressing change to be $94.01 per day,36 so reducing the frequency of these dressing changes from 3 times per week to once per week is not insignificant. The use of ECM products adjunctively with NPWT to synergistically improve wound healing trajectory has been widely described in the scientific literature.37-39 It has been postulated previously that collagen-based ECM products used concurrently with NPWT can enhance wound healing properties and shorten the duration of NPWT use.40
As previously discussed, the use of certain dermal matrices, including synthetic matrices, may result in higher rates of infection when placed in contaminated fields.41,42 Infection rates seen for synthetic dermal matrices have been reported as high as 20%, 29.6%, and 18.1% in lower extremity reconstructions,31 burns,43 and trauma,44 respectively. While methods to “milk” infection from these devices have been reported,31 rates of graft loss as high as 20% have been reported in cases where this intervention was unsuccessful.31 OFM is an intact ECM that is minimally processed to remove the ovine cells while maintaining ECM structure45 and biology46 with no chemical cross-linking that may otherwise inhibit the rate of neovascularization47 and induce a proinflammatory tissue response.48 In contrast to reports for synthetic dermal matrices,42 we observed no infections in the current study, leading us to conclude that OFM is relatively resistant to infection and sufficiently robust to perform in a contaminated field.
Limitations
The current pilot study comprises observations from a single center with all the limitations of a retrospective case series. Not surprisingly, several of the patients included in the current report were lost to follow-up before complete closure of the volumetric defect (Table 2). This outcome, unfortunately, is entirely consistent with the high follow-up failure rate of trauma patients due to lack of insurance coverage, discharge to tertiary care facilities, and lack of patient education regarding the importance of follow-up care.49-51 While the results of this case series are promising, there is a need for future research to expand the number of patients to validate these initial results. Future studies may involve a controlled study design, but this may be complicated by the absence of a “gold-standard” for the reconstruction of volumetric soft tissue defects.
Conclusions
This retrospective pilot case series builds on a growing body of evidence that OFM can be utilized to facilitate the formation of functional, well-vascularized soft tissue in large, contaminated, volumetric soft tissue defects. The OFM grafts were shown to complement NPWT and may reduce the frequency of dressing changes associated with NPWT usage in these complex soft reconstructive procedures.
Acknowledgments
The authors whish to acknowledge Aroa Biosurgery Limited for editorial assistance in preparing this manuscript. We thank the efforts of the nurses and surgical team of Northeast Georgia Medical Center.
Affiliations: 1Northeast Georgia Medical Center, Department of Trauma and Acute Care Surgery, Gainesville, Georgia; 2Aroa Biosurgery Limited, Auckland, New Zealand; 3Northeast Georgia Medical Center, Northeast Georgia Physicians Group Vascular Center, Gainesville, Georgia
Correspondence: William M Vassy, MD; docvassy@icloud.com
Ethics: WCG Clinical provided institutional review board (IRB) services under work order 1-1572217-1. IRB approval was obtained on August 3, 2022. All patients provided written informed consent for their images and data to be used for research and publication purposes.
Disclosures: BAB and SGD are employees of Aroa Biosurgery Limited. WMV and MTC have received honoraria from Aroa Biosurgery Limited. No direct funding was received for this study.
References
1. Guest JF, Fuller GW, Vowden P. Costs and outcomes in evaluating management of unhealed surgical wounds in the community in clinical practice in the UK: a cohort study. BMJ Open. 2018;8(12):e022591. doi:10.1136/bmjopen-2018-022591
2. Flood MS, Weeks B, Anaeme KO, et al. Treatment of deep full-thickness wounds containing exposed muscle, tendon, and/or bone using a bioactive human skin allograft: a large cohort case series. Wounds. 2020;32(6):164-173.
3. Grogan BF, Hsu JR, Skeletal Trauma Research C. Volumetric muscle loss. J Am Acad Orthop Surg. 2011;19 Suppl 1:S35-7. doi:10.5435/00124635-201102001-00007
4. Sapino G, Lanz L, Roesti A, et al. One-stage coverage of leg region defects with STSG combined with VAC dressing improves early patient mobilisation and graft take: a comparative study. J Clin Med. 2022;11(12)doi:10.3390/jcm11123305
5. Sams HH, McDonald MA, Stasko T. Useful adjuncts to harvest split-thickness skin grafts. Dermatol Surg. 2004;30(12 Pt 2):1591-2. doi:10.1111/j.1524-4725.2004.30573.x
6. Um JH, Jo DI, Kim SH. New proposal for skin grafts on tendon-exposed wounds. Arch Plast Surg. 2022;49(1):86-90. doi:10.5999/aps.2021.00297
7. Kanchwala SK, Glatt BS, Conant EF, Bucky LP. Autologous fat grafting to the reconstructed breast: the management of acquired contour deformities. Plast Reconstr Surg. 2009;124(2):409-418. doi:10.1097/PRS.0b013e3181aeeadd
8. Kim TG, Choi MK. Secondary contouring of flaps. Arch Plast Surg. 2018;45(4):319-324. doi:10.5999/aps.2018.00542
9. Saber AY, Hohman MH, Dreyer MA. Basic flap design. Updated August 29, 2022. Accessed August 14, 2023. StatPearls. https://www.ncbi.nlm.nih.gov/books/NBK563252/
10. Zeiderman MR, Pu LLQ. Contemporary approach to soft-tissue reconstruction of the lower extremity after trauma. Burns Trauma. 2021;9:tkab024. doi:10.1093/burnst/tkab024
11. Schneeberger SJ, Kraft CT, Janis JE. No-touch technique of mesh placement in ventral hernia repair: minimizing postoperative mesh infections. Plast Reconstr Surg. 2020;145(5):1288-1291. doi:10.1097/PRS.0000000000006767
12. Cramer MC, Badylak SF. Extracellular matrix-based biomaterials and their influence upon cell behavior. Ann Biomed Eng. 2020;48(7):2132-2153. doi:10.1007/s10439-019-02408-9
13. Ousey KD, Djohan R, Dowsett C, et al. World Union of Wound Healing Societies consensus document. Surgical wound dehiscence: improving prevention and outcomes. February 20, 2018. Accessed August 14, 2023. Wounds UK. https://huddersfield.box.com/s/gw7grmclaxctndsuan3aydwt2qdkoown
14. Chaffin AE, Buckley MC. Extracellular matrix graft for the surgical management of Hurley stage III hidradenitis suppurativa: a pilot case series. J Wound Care. 2020;29(11):624-630. doi:10.12968/jowc.2020.29.11.624
15. Chaffin AE, Dowling SG, Kosyk MS, Bosque BA. Surgical reconstruction of pilonidal sinus disease with concomitant extracellular matrix graft placement: a case series. J Wound Care. 2021;30(Sup7):S28-S34. doi:10.12968/jowc.2021.30.Sup7.S28
16. Bosque BA, Frampton C, Chaffin AE, et al. Retrospective real-world comparative effectiveness of ovine forestomach matrix and collagen/ORC in the treatment of diabetic foot ulcers. Int Wound J. 2022;19(4):741-753. doi:10.1111/iwj.13670
17. Miller DL, Durden FL. Chest wall reconstruction utilizing ovine-derived reinforced tissue matrix. Ann Thorac Surg. 2023 May;115(5):1266-1272. doi:10.1016/j.athoracsur.2021.12.062
18. Ferzoco SJ. Early experience outcome of a reinforced Bioscaffold in inguinal hernia repair: a case series. Int J Surg Open. 2018;12:9-11. doi:10.1016/j.ijso.2018.06.001
19. Sawyer MAJ. New ovine polymer-reinforced bioscaffold in hiatal hernia repair. JSLS. Oct-Dec 2018;22(4): e2018.00057. doi:10.4293/JSLS.2018.00057
20. DeNoto G, Ceppa EP, Pacella SJ, et al. A prospective, single arm, multi-center study evaluating the clinical outcomes of ventral hernias treated with OviTex® 1S permanent reinforced tissue matrix: the BRAVO study 12-month analysis. J Clin Med. 2021;10(21):4998. doi:https://doi.org/10.3390/jcm10214998
21. Ankney C, Banaschak C, Sowers B, Szotek P. Minimizing retained foreign body in hernia repair using a novel technique: reinforced biologic augmented repair (ReBAR). J Clin Med Res. 2021;3(4):1-11. doi:https://doi.org/10.37191/Mapsci-2582-4333-3(4)-073
22. Sivaraj D, Henn D, Fischer KS, et al. Reinforced biologic mesh reduces postoperative complications compared to biologic mesh after ventral hernia repair. Plast Reconstr Surg Glob Open. Feb 2022;10(2):e4083. doi:10.1097/GOX.0000000000004083
23. DeNoto G. Bridged repair of large ventral hernia defects using an ovine reinforced biologic: a case series. Ann Med Surg (Lond). 2022;75:103446. doi:https://doi.org/10.1016/j.amsu.2022.103446
24. Timmer AS, Claessen JJM, Brouwer de Koning IM, et al. Clinical outcomes of open abdominal wall reconstruction with the use of a polypropylene reinforced tissue matrix: a multicenter retrospective study. Hernia. 2022;26(5):1241-1250. doi:10.1007/s10029-022-02604-y
25. Overbeck N, Nagvajara GM, Ferzoco S, May BCH, Beierschmitt A, Qi S. In-vivo evaluation of a reinforced ovine biologic: a comparative study to available hernia mesh repair materials. Hernia. 2020;24(6):1293-1306. doi:10.1007/s10029-019-02119-z
26. Negron L, Lun S, May BCH. Ovine forestomach matrix biomaterial is a broad spectrum inhibitor of matrix metalloproteinases and neutrophil elastase. Int Wound J. 2012;11(4):392-397.
27. Zhao R, Liang H, Clarke E, Jackson C, Xue M. Inflammation in chronic wounds. Int J Mol Sci. 2016;17(12):2085. doi:10.3390/ijms17122085
28. Li D, Wang C, Wei W, et al. Postoperative complications of free flap reconstruction in moderate-advanced head and neck squamous cell carcinoma: a prospective cohort study based on real-world data. Front Oncol. 2022;12:792462. doi:10.3389/fonc.2022.792462
29. Yannas IV. Tissue regeneration by use of collagen-glycosaminoglycan copolymers. Clin Mater. 1992;9(3-4):179-187. doi:10.1016/0267-6605(92)90098-e
30. Yannas IV, Burke JF. Design of an artificial skin. I. Basic design principles. J Biomed Mater Res. 1980;14(1):65-81. doi:10.1002/jbm.820140108
31. Solanki NS, York B, Gao Y, Baker P, Wong She RB. A consecutive case series of defects reconstructed using NovoSorb Biodegradable Temporising Matrix: initial experience and early results. J Plast Reconstr Aesthet Surg. 2020;73(10):1845-1853. doi:10.1016/j.bjps.2020.05.067
32. Bohn GA, Chaffin AE. Extracellular matrix graft for reconstruction over exposed structures: a pilot case series. J Wound Care. 2020;29(12):742-749. doi:10.12968/jowc.2020.29.12.742
33. Stone R, 2nd, Saathoff EC, Larson DA, et al. Accelerated wound closure of deep partial thickness burns with acellular fish skin graft. Int J Mol Sci. 2021;22(4):1590. doi:10.3390/ijms22041590
34. Schlanser V, Dennis A, Ivkovic K, et al. Placenta to the rescue: limb salvage using dehydrated human amnion/chorion membrane. J Burn Care Res. 2018;39(6):1048-1052. doi:10.1093/jbcr/irx031
35. Norman G, Goh EL, Dumville JC, et al. Negative pressure wound therapy for surgical wounds healing by primary closure. Cochrane Database Syst Rev. 2020;6:CD009261. doi:10.1002/14651858.CD009261.pub6
36. Kim JJ, Franczyk M, Gottlieb LJ, Song DH. Cost-effective alternative for negative-pressure wound therapy. Plast Reconstr Surg Glob Open. 2017;5(2):e1211. doi:10.1097/GOX.0000000000001211
37. Fleming ME, O’Daniel A, Bharmal H, Valerio I. Application of the orthoplastic reconstructive ladder to preserve lower extremity amputation length. Ann Plast Surg. 2014;73(2):183-9. doi:10.1097/SAP.0b013e3182a638d8
38. Dillingham CS, Jorizzo J. Managing ulcers associated with pyoderma gangrenosum with a urinary bladder matrix and negative-pressure wound therapy. Adv Skin Wound Care. Feb 2019;32(2):70-76. doi:10.1097/01.ASW.0000546120.32681.bc
39. Gabriel A, Gollin G. Management of complicated gastroschisis with porcine small intestinal submucosa and negative pressure wound therapy. J Pediatr Surg. 2006;41(11):1836-1840. doi:10.1016/j.jpedsurg.2006.06.050
40. Sreelesh LS, Laxminarayan Bhandari P. An easy technique for negative-pressure wound therapy for extremities using collagen powder and sterile gloves. Indian J Surg. 2017;79(1):81-83. doi:10.1007/s12262-017-1589-z
41. Li H, Lim P, Stanley E, et al. Experience with NovoSorb® Biodegradable Temporising Matrix in reconstruction of complex wounds. ANZ Journal of Surgery. 2021;91(9):1744-1750. doi:10.1111/ans.16936
42. Gonzalez SR, Wolter KG, Yuen JC. Infectious complications associated with the use of Integra: a systematic review of the literature. Plast Reconstr Surg Glob Open. 2020;8(7):e2869. doi:10.1097/GOX.0000000000002869
43. Bargues L, Boyer S, Leclerc T, Duhamel P, Bey E. [Incidence and microbiology of infectious complications with the use of artificial skin Integra in burns]. Ann Chir Plast Esthet. Dec 2009;54(6):533-539. doi:10.1016/j.anplas.2008.10.013
44. Huang SR, Liu JT, Zhang Y, Ouyang RL, Ruan MZ, Luo B. [Repair of complex wounds on hands after burns or trauma]. Zhonghua Shao Shang Za Zhi. 2019;35(5):362-366. doi:10.3760/cma.j.issn.1009-2587.2019.05.007
45. Sizeland KH, Wells HC, Kelly SJR, et al. Collagen fibril response to strain in scaffolds from ovine forestomach for tissue engineering. ACS Biomater Sci Eng. 2017;3(10):2550-2558. doi:10.1021/acsbiomaterials.7b00588
46. Dempsey SG, Miller CH, Hill RC, Hansen KC, May BCH. Functional insights from the proteomic inventory of ovine forestomach matrix. J Proteome Res. 2019;18(4):1657-1668. doi:10.1021/acs.jproteome.8b00908
47. Mestak O, Spurkova Z, Benkova K, et al. Comparison of cross-linked and non-cross-linked acellular porcine dermal scaffolds for long-term full-thickness hernia repair in a small animal model. Eplasty. 2014;14:e22.
48. Delgado LM, Bayon Y, Pandit A, Zeugolis DI. To cross-link or not to cross-link? Cross-linking associated foreign body response of collagen-based devices. Tissue Eng Part B Rev. 2015;21(3):298-313. doi:10.1089/ten.TEB.2014.0290
49. Stone ME, Jr., Marsh J, Cucuzzo J, Reddy SH, Teperman S, Kaban JM. Factors associated with trauma clinic follow-up compliance after discharge: experience at an urban Level I trauma center. J Trauma Acute Care Surg. 2014;76(1):185-190. doi:10.1097/TA.0b013e3182aafcd5
50. Overton TL, Shafi S, Gandhi RR. Local access to care programs increase trauma patient follow-up compliance. Am J Surg. 2014;208(3):476-479. doi:10.1016/j.amjsurg.2013.11.008
51. Leukhardt WH, Golob JF, McCoy AM, Fadlalla AM, Malangoni MA, Claridge JA. Follow-up disparities after trauma: a real problem for outcomes research. Am J Surg. 2010;199(3):348-352; discussion 353. doi:10.1016/j.amjsurg.2009.09.021